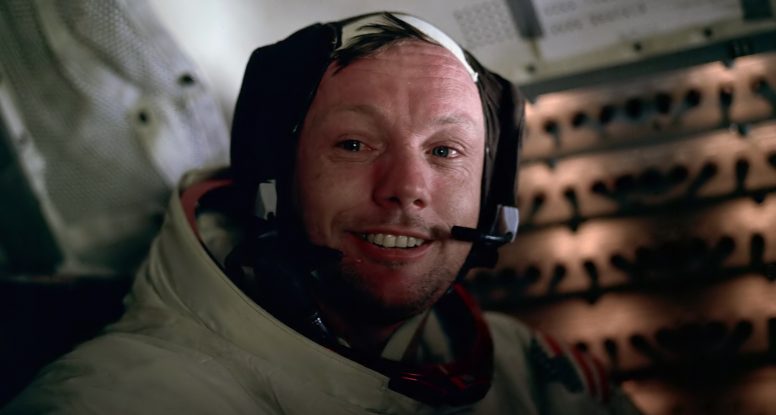
How Did We Do It?
An Internet denizen asks:
Q: How were we able to put a man on the moon with the level of technology that was available in 1969?
The answer? By spending a crap ton of money over ten years, peaking at 5% of the federal budget. And by relying as much at possible on already proven technology, which isn’t as “high-tech” as you think (more on that below).
Q: Did NASA have advanced technologies that were just not made public?
Very little. They developed the advanced technologies they needed, and except where they were borrowing from the military, they then made them public. NASA’s primary job, after all, is to promote and nurture the American aerospace industry.
Here are a few examples of how the technology came to be:
- Back in 1961, NASA knew it would need a big moon rocket, but they didn’t know how big or have a design for it. They knew, however, that back in 1955, Rocketdyne had started work on the granddaddy of rocket engines for the Air Force. The first attempt (the E-1) had been a dead end, but the second try (the F-1) had been successfully fired in 1957—the year before NASA was founded. The Air Force had abandoned the engine, but NASA paid Rocketdyne to continue development, and the engine was improved continually throughout the Apollo program, including thrust and reliability upgrades from one mission to another. For all that, the F-1 was in many ways a crude engine by today’s standards. In particular, it required hundreds of difficult, manual welds in refractory metal, which all had to be perfect. Today, the same engine could be formed in three (principle) pieces and welded together by robots, but back then, it was all done by hand.
- NASA knew the big moon rocket would need an utterly reliable navigation system—though they didn’t know how good it really needed to be. They also knew it would need a more flexible computer than missiles of the time, or the crew would be overwhelmed. The MIT Instrumentation Laboratory had been involved in both fields for the military, so it was the obvious choice to design the IMU, Computer, and navigational sensors. Companies like Raytheon, who had built earlier systems for the military, were the obvious people to build it. It turned out that radio ranging from Earth was about as accurate as the Apollo navigation system, but the crew were happy to have the backup. For the most part, simple sailing tools would have been sufficient. Again, this bleeding edge 1969 technology is mickey mouse today. A modern laser ring gyroscope is more accurate, less power hungry, and a damn site lighter than the Apollo IMU, and my cell phone has 100,000 times the power of the 70 pound, 55 watt AGC. NASA did, however, buy integrated circuits as fast as Fairchild Semiconductor could make them, spurring development in the field, and they had to learn to build human-rated systems for the radiation filled space environment and keep them cool in a cabin that had no air convection (and sometimes no air), and we still use that “technology” today.
- Since the new the moon rocket was going to take a while (whether the Nova or the later Saturn form) Werner Von Braun’s team assembled a Frankenstein’s Erector Set of spare parts from Juno, Jupiter, and other earlier work, and while bizarre and not very efficient, the resultant “Saturn I” served Apollo’s needs in low Earth orbit through the Skylab era.
- When Boeing finally started on the Saturn, they found that the first stage required aluminum welds longer than anyone in the world knew how to make. So they figured it out. Today, we can weld nuclear submarine hulls.
- When North American Aviation was given the contract for the Saturn second stage (The S-II) they didn’t have enough wight budget for the normal bulkheads and insulate between the liquid oxygen and hydrogen tanks. They came up with a common bulkhead made of fiberglass and phenolic resin. When they had trouble getting the (then cutting edge) epoxy adhesives to perform, they called on local surf board makers who were happy to share their expertise (That might have been the S-IVB upper stage, built by Douglas).
- To study propellant behavior in large booster stages, NASA borrowed ten-year old technology from early spy satellites and used ejectable pods to recover film cameras mounted inside the tanks.
- To save power, the Command Module was primarily lit by bespoke compact fluorescent floodlights, made by GE using technology already under development, and later refined into the (now essentially obsolete) CFL.
- LEM design started in 1962 with a request for proposals to 11 firms. As development of the rest of the mission components proceeded, however, every limitation or compromise ate into the allowable weight for the LEM. Later improvements in engine thrust and operational efficiency opened that up and enabled the heavier, later missions, but when Grumman won the contract, the weight restrictions seemed impossible to meet. So they ditched the chairs (who need’s ‘em?) and the windows (portals will do, if facing just right). They held company-wide contests to shave weight off the design. For the pressure hull/fuselage, they machined solid sheets of aircraft aluminum into grids, then chemically etched the remaining metal to create tapered panels, barely thicker than a soda can in some places, but sufficient to hold the mere 5 psi atmosphere within the LEM. It worked and met NASA’s requirements, but it cost a fortune, and each LEM was rated for only a few pressurization cycles. Today, we could build the ascent stage for half the weight and 100 times the life using carbon fiber composits, and cut the descent stage (dry) weight by about 80%.
- Large booster stages could rely on acceleration (either from the main engines or small “ullage motors” to keep propellants flowing, but that wouldn’t work for true spacecraft, which needed to be able to fire thrusters at will, both for ullage and maneuvering, and as a backup to main engine failure. NASA’s solution was to use rubber bladders like those used in WWII aircraft to prevent fuel tanks from exploding when hit by enemy fire. Helium, pressing between the bladder and the intake, kept the propellant where it needed to be. You sometimes hear people talking about how the Apollo ascent engine couldn’t be tested because the hypergolic propellants would have corroded it before launch. That’s not true. The engine (and thrusters) could easily be made to resist corrosion, but the rubber bladders couldn’t. When the space shuttle cam allong, NASA spent a lot of money inventing an alternative that relies on surface tension and operational restrictions to eliminate the need for the bladder (or a more complex alternative), and that’s why the shuttle’s OMS engines and thrusters didn’t have to be replaced after every flight.
- The main heat shield for the first 6 Mercury flights was a single piece of machined beryllium—a technology lifted from earlier missiles. Mercury and Gemini in turn were protected from reentry heating by high temperature alloy shingles developed from research into nuclear missiles. The heat shield for the Apollo spacecraft was derived from the ablative main shield used in Gemini and later Mercury flights. The heat shield for the leading edges of the space shuttle was derived from research into the ablative shields used on Apollo. The ablative heat shield used by the modern Orion, Space-x and Boeing capsules was developed from that used on Apollo, modified to essentially decompose into the pyrolyzed carbon leading edges from the shuttle, creating a reusable, self-renewing shield.
By the way. NASA wasn’t created to send people to the moon. It was created to assist American aerospace and in matters of aeronautical or space, the American public and all mankind.
The reason we could build a LEM of composites today…the reason Boeing can build a jumbo jet of composites today—is in part because NASA paid to have those materials characterized so they could use them on the payload bay doors of the shuttle. And the reason today’s space entreprenuers can show of their fancy propulsive landing systems is—in large part—because NASA paid a lot of money to essentially create the practice of Computational Fluid Dynamics, without which neither the shuttle nor these modern wonders could have flown.
We went to the moon with what was, at the time, cutting edge, custom-made, sometimes poorly understood, often barely adequate technology. The basic science hasn’t changed must since, but our manufacturing ability has grown by leaps and bounds—in part due to the spur it received from Apollo and the last fifty years of refinement.
Great information.Thank you.